ISSCA to Conduct Regenerative Medicine Conference in Cancún, México
Miami, FL, December 19, 2022 – The International Association for Stem Cell Application (ISSCA) has announced plans to host Applications of Stem Cell Therapies in Medicine and Aesthetic Surgery, a regenerative medicine conference, in Cancun, Mexico, April 22, 23, and 24, 2023.
Growing knowledge can turn the tide in the clinical and translational spectrum of stem-cell-based research and therapy, and several obstacles must be overcome. ISSCA’s International Conference, champions new ideas to propel the shift from traditional healthcare to regenerative medicine and therapies. The Conference will also emphasize the role of cutting technology and developments in all areas of stem cell research.
April 22nd & 23rd
The Cancun event will feature a panel of internationally renowned experts on stem cells and regenerative medicine, who will offer a two-day rigorous scientific discourse on critical topics in stem cell research and clinical applications. Topics of focus at the conference include: Tissue engineering and regenerative medicine; updates in Stem Cell research Clinical applications of regenerative medicine and cellular therapies; the latest methods of harvest and isolation, and Non-invasive surgical protocols; strategies for successful delivery of cell products; Ethical and regulatory issues in stem cell technology; and taking advantage of the latest technologies to improve marketing and networking.
April 24th
The third day of the conference (April 24th) will involve intensive hands-on training sessions with ISSCA-certified instructors on stem cell therapy’s aesthetic and clinical applications.
Participants will gain experience and access to different technologies, brands, and products for stem cell therapy.
The leading international conference offers an excellent opportunity for medical practitioners and stakeholders to network and learn from the best in the industry.
All practitioners and stakeholders in the medical sector are invited, including:
- Doctors Researchers & innovators,
- Decision-makers and funding agencies,
- Experts seeking collaborative work on stem cell technology,
- Professional bodies, and
- Marketing professionals.
To learn more about the ISSCA Cancun, Mexico conference and to make a reservation, visit the https://www.issca.us/issca-world-conferences/ website, email info@stemcellsgroup.com, or call +1305 560 5337.
About ISSCA
The International Society for Stem Cell Application (ISSCA) is a multidisciplinary community of scientists and physicians who aspire to treat diseases and lessen human suffering through advances in science, technology, and regenerative medicine.
ISSCA updates its members on advances in stem cell research, MSC, exosomes, and regenerative medicine.
The ISSCA’s vision is to take a leadership position in promoting excellence and setting standards in the regenerative medicine fields of publication, research, education, training, and certification.
The international community provides a platform for practitioners to interact with scientists and build medical networks necessary for marketing.
As a medical specialty, regenerative medicine standards and certifications are essential, which is why ISSCA offers certification training in cities worldwide. The goal is to encourage more physicians to practice regenerative medicine and make it available to patients nationally and internationally. Incorporated under the Republic of Korea as a non-profit company, the ISSCA is focused on fostering excellence and standards in regenerative medicine.
About Global Stem Cells Group
Global Stem Cells Group is the parent company of six companies that are dedicated to stem cell research, solutions, and technology training. The group was founded in 2012 and combines dedicated researchers, patient educators, and physician trainers with the shared goal of meeting the need for high-end stem cell solutions and treatments.
Given that the group has a singular focus in this field, it is uniquely positioned to become the global leader in cellular medicine. In addition, by bringing together leading professionals in cellular medicine, it can discover issues that the industry faces and focus its research and development in this area. This specialization has, undoubtedly, enabled it to come up with solutions that address some of the significant issues that most stakeholders are facing in the industry.
Global Stem Cells Group is a publicly traded company operating under the symbol MSSV. https://finance.yahoo.com/quote/mssv/
- Published in Press Releases
A federal judge recently ruled in favor of a regenerative medicine clinic against the FDA
The FDA brought the lawsuit against Cell Surgical Network Clinic in 2018 in an attempt to assert regulatory authority over stem cell therapies. The agency argued that a physician’s use of his patient’s own stem cells as part of the medical treatment provided to the patient was equivalent to manufacturing a biological drug product and therefore subject to regulation by the FDA.
The court rejected this argument on Tuesday, ruling that the surgical procedure does not create a new prescription drug. The court wrote: “The adipose tissue Defendants remove from patients clearly consists of human cells. And whatever is injected back into patients as part of Defendants’ SVF Surgical Procedure and Expanded MSC Surgical Procedure certainly contains such cells.”
This news opens up the possibilities and sets an important precedent for clinics in the United States to use autologous cellular treatments derived from adipose tissue, giving patients the right to use their own tissue to enhance regenerative processes and fight disease.
“We appreciate the Court’s clear and unequivocal ruling, which affirms what we have been saying for 12 years: that our innovative surgical approach to personal cell therapy is safe and legal,” said Dr. Elliott Lander, co-founder of CSCTC. “With this victory behind us, we look forward to refocusing our energy on our practice and leveraging life-changing stem cell treatments to support physicians and benefit patients across the country.”
As science advances, it’s inevitable that there will be more diverse forms of treatment with human stem cells. Whether the FDA gets on board and expands its rules about what is and is not legal is another question.
Unfortunately, much of what the FDA does falls into the category of politics. It’s usually unwise to make a decision about a particular kind of therapy or medical treatment based on whether it’s technically, currently legal.
It’s helpful to recall that many treatments that are fully legal began as experimental procedures that were not legally offered to the public but only took place in controlled research environments.
The good news is that stem cell therapy continues to make rapid advances on several different fronts, primarily those related to joint pain and associated discomfort. Athletes have made good use of the treatment and were, in fact, among the first major wave of clients for the technique when it was still brand new. After that, word spread about the effectiveness of stem cell treatment as more and more people turned to it as an alternative to surgery and prescription medication.
- Published in Press Releases
Stem cell treatment could offer one-end-solution to Diabetes
Insulin-producing cells grown in the lab could provide a possible cure for the age-long disease (diabetes).
Type 1 diabetes is an auto¬immune disease that wipes out insulin-producing pancreatic beta cells from the body and raises blood glucose to dangerously high levels. These high levels of Blood sugar level can be even fatal. Patients are being administered insulin and given other medications to maintain blood sugar level. To those who cannot maintain their blood sugar level, they are given beta-cell transplants but to tolerate beta cell transplants; patients have to take immunosuppressive drugs as well.
A report by a research group at Harvard University tells us that they used insulin-producing cells derived from human embryonic stem cells (ESCs) and induced pluripotent stem cells to lower blood glucose levels in mice. Nowadays, many laboratories are getting rapid progress in human stem cell technology to develop those cells that are functionally equivalent to beta-cells and the other pancreatic cell types. Other groups are developing novel biomaterials to encapsulate such cells and protect them against the immune system without the need for immunosuppressant.
Major pharmaceutical companies and life sciences venture capital firms have invested more than $100 million in each of the three most prominent biotechnological industries to bring such treatments into clinical use:
- Cambridge
- Massachusetts–based companies Semma Therapeutics
- Sigilon Therapeutics, and ViaCyte of San Diego
Researchers of UC San Francisco have transformed human stem cells into mature insulin-producing cells for the first time, a breakthrough in the effort to develop a cure for type-1 (T1) Diabetes. Replacing these cells, which are lost in patients with T1 diabetes, has long been a dream of regenerative medicine, but until now scientists had not been able to find out how to produce cells in a lab dish that work as they do in healthy adults.
What is T1 diabetes?
T1 diabetes is an autoimmune disorder that destroys the insulin-producing beta cells of the pancreas, typically in childhood. Without insulin’s ability to regulate glucose levels in the blood, spikes in blood sugar can cause severe organ damage and eventually death. The condition can be managed by taking regular shots of insulin with meals. However, people with type 1 diabetes still often experience serious health consequences like kidney failure, heart disease and stroke. Patients facing life-threatening complications of their condition may be eligible for a pancreas transplant from a deceased donor, but these are rare, and they are supposed to wait a long time.
Researchers have just made a breakthrough that might one day make these technologies obsolete, by transforming human stem cells into functional insulin-producing cells (also known as beta cells) – at least in mice.
“We can now generate insulin-producing cells that look and act a lot like the pancreatic beta cells you and I have in our bodies,” explains one of the team, Matthias Hebrok from the University of California San Francisco (UCSF).
“This is a critical step towards our goal of creating cells that could be transplanted into patients with diabetes.”
Type-1 diabetes is characterized by a loss of insulin due to the immune system destroying cells in the pancreas – hence, type 1 diabetics need to introduce their insulin manually. Although this is a pretty good system, it’s not perfect.
Making insulin-producing cells from stem cells
Diabetes can be cured through an entire pancreas transplant or the transplantation of donor cells that produce insulin, but both of these options are limited because they rely on deceased donors. Scientists had already succeeded in turning stem cells into beta cells, but those cells remained stuck at an early stage in their maturity. That meant they weren’t responsive to blood glucose and weren’t able to secrete insulin in the right way.
Scientists at the University of California San Francisco made a breakthrough in the effort to cure diabetes mellitus type 1.
For the first time, researchers transformed human stem cells into mature insulin-producing cells, which could replace those lost in patients with the autoimmune. There is currently no known way to prevent type-1 (T1) diabetes, which destroys insulin production in the pancreas, limits glucose regulation, and results in high blood sugar levels. The condition can be managed with regular shots of insulin, but people with the disease often experience serious health complications like kidney failure, heart disease, and stroke.
“We can now generate insulin-producing cells that look and act a lot like the pancreatic beta cells you and I have in our bodies,” according to Matthias Hebrok, senior author of a study published last week in the journal Nature Cell Biology.
“This is a critical step toward our goal of creating cells that could be transplanted into patients with diabetes,” Hebrok, director of the UCSF Diabetes Center, said in a statement.
Islets of Langerhans are groupings of cells that contain healthy beta cells, among others. As beta cells develop, they have to separate physically from the pancreas to form these islets.
The team artificially separated the pancreatic stem cells and regrouped them into these islet clusters. When they did this, the cells matured rapidly and become responsive to blood sugar. In fact, the islet clusters developed in ways “never before seen” in a lab. After producing these mature cells, the team transplanted them into mice. Within days, the cells were producing insulin similar to the islets in the mice. While the study has been successful in mice, it still needs to go through more rigorous testing to see if it would work for humans as well. But the research is up-and-coming. “We can now generate insulin-producing cells that look and act a lot like the pancreatic beta cells you and I have in our bodies. This is a critical step towards our goal of creating cells that could be transplanted into patients with diabetes,” He said.
“We’re finally able to move forward on several different fronts that were previously closed to us,” he added. “The possibilities seem endless.”
Basic research keeps elucidating new aspects of beta cells; there seem to be several subtypes, so the gold standard for duplicating the cells is not entirely clear. Today, however, there is “a handful of groups in the world that can generate a cell that looks like a beta cell,” says Hebrok, who currently acts as scientific advisor to Semma and Sigilon, and has previously advised ViaCyte. “Certainly, companies have convinced themselves that what they have achieved is good enough to go into patients.”
The stem cell reprogramming methods that the three companies use to prompt cell differentiation create a mixture of islet cells. Beta cells sit in pancreatic islets of Langerhans alongside other types of endocrine cells. Alpha cells, for example, churn out glucagon, a hormone that stimulates the conversion of glycogen into glucose in the liver and raises blood sugar. Although the companies agree on the positive potential of islet cell mixtures, they take different approaches to developing and differentiating their cells. Semma, which was launched in 2014 to commercialize the Harvard group’s work and counts Novartis among its backers, describes its cells as fully mature, meaning that they are wholly differentiated into beta or other cells before transplantation. “Our cells are virtually indistinguishable from the ones you would isolate from donors,” says Semma chief executive officer BastianoSanna
To get around the donor problem, researchers, including the team at UCSF has been working on nudging stem cells into becoming fully-functional pancreatic beta cells for the last few years. Still, there have been some issues in getting them all the way there.
“The cells we and others were producing were getting stuck at an immature stage where they weren’t able to respond adequately to blood glucose and secrete insulin properly,” Hebrok said.
“It has been a major bottleneck for the field.”
“We’re finally able to move forward on a number of different fronts that were previously closed to us,” Hebrok added. “The possibilities seem endless.”
Regardless of starting cell type, the companies say they are ready to churn out their cells in large numbers. Semma, for example, can make more islet cells in a month than can be isolated from donors in a year in the United States, Sanna says, and the company’s “pristine” cells should perform better than donor islets, which are battered by the aggressive techniques required for their isolation.
As these products, some of which have already entered clinical trials, move toward commercialization, regulatory agencies such as the US Food and Drug Administration (FDA) and the European Medicines Agency have expressed concern about the plasticity of the reprogrammed cells. All three firms subject their cells to rigorous safety testing to ensure that they don’t turn tumorigenic. Before successful trials, companies won’t know the dose of beta cells required for a functional cure, or how long such “cures” will last before needing to be boosted. There’ll be commercial challenges, too: while the companies are investing heavily to develop suitable industrial processes, all acknowledge that no organization has yet manufactured cell therapies in commercial volumes.
Nevertheless, there’s growing confidence throughout the field that these problems will be solved, and soon. “We have the islet cells now,” says Alice Tomei, a biomedical engineer at the University of Miami who directs DRI’s Islet Immuno-engineering Laboratory.
“These stem cell companies are working hard to try to get FDA clearance on the cells.”
Protecting stem cell therapies from the immune system
Whatever the type of cell being used, another major challenge is delivering cells to the patient in a package that guards against immune attack while keeping cells fully functional. Companies are pursuing two main strategies:
- Microencapsulation, where cells are immobilized individually or as small clusters, in tiny blobs of a biocompatible gel.
- Macroencapsulation, in which greater numbers of cells are put into a much larger, implantable device.
ViaCyte, which recently partnered with Johnson & Johnson, launched its first clinical trial in 2014. The trial involved a micro-encapsulation approach that packaged up the company’s partially differentiated, ESC-derived cells into a flat device called the PEC-Encapsulation. About the size of a Band-Aid, the device is implanted under the skin, where the body forms blood vessels around it. “It has a semipermeable membrane that allows the free flow of oxygen, nutrients, and glucose,” says ViaCyte’s chief executive officer, Paul Laikind. “And even proteins like insulin and glucagon can move back and forth across that membrane, but cells cannot.”
The trial showed that the device was safe, well-tolerated, and protected from the adaptive immune system—and that some cells differentiated into working islet cells. But most cells didn’t engraft effectively because a “foreign body response,” a variant of wound healing, clogged the PEC-Encap’s membrane and prevented vascularization. ViaCyte stopped the trial and partnered with W. L. Gore & Associates, the maker of Gore-Tex, to engineer a new membrane. “With this new membrane,” says Laikind, “we’re not eliminating that foreign body response, but we’re overcoming it in such a way that allows vascularization to take place.” The company expects to resume the trial in the second half of this year, provided it receives the green light from the FDA.
Semma is also developing macro¬-encapsulation methods, including a very thin device that in prototype form is about the size of a silver dollar coin. The device is “deceptively simple, but it allows us to put [in] a fully curative dose of islets,” Sanna says.
Semma is also investigating microencapsulation alternatives. At the same time, the company is advancing toward clinical trials using established transplantation techniques to administer donated cadaver cells to high-risk patients who find it particularly difficult to control their blood glucose levels. These cells are infused via the portal vein into the liver, and patients take immunosuppressive drugs to prevent rejection.
Sigilon is working on its microencapsulation technology. Launched in 2016 on the back of work by the labs of Robert Langer and Daniel Anderson at MIT, the company has created 1.5-millimeter gel-based spheres that can hold between 5,000 and 30,000 cells (Nat Med, 22:306–11, 2016). Each sphere is like a balloon, with the outside chemically modified to provide immune-protection, says Sigilon chief executive officer Rogerio Vivaldi. “The inside of the balloon is full of a gel that creates almost a kind of a matrix net where the cells reside.”
In 2018, shortly after partnering with Eli Lilly, Sigilon and collaborators published research showing that islet cells that were encapsulated in gel spheres and transplanted into macaques remained functional for four months. The company has not disclosed a time frame for a type 1 diabetes trial “but we’re moving pretty quickly,” says chief scientific officer David Moller.
Conclusion
To conclude, all three firms hope to extend their work to treat some of the 400 million people worldwide with type 2 diabetes, many of them eventually benefit from insulin injections. The recent endorsements from big Pharmaceutical underline the real progress in beta-cell transplants, says Aaron Kowalski, a molecular geneticist and chief executive officer at JDRF, a foundation based in New York that has funded research at ViaCyte and academic labs whose work has been tapped by Semma and Sigilon. “These companies all realize that if they don’t do it, somebody else will. It’s hard to predict exactly when, but somebody is going to make this work.”
- Published in Corporate News / Blog
Stem cell treatment could offer one-end-solution to Diabetes
Insulin-producing cells grown in the lab could provide a possible cure for the age-long disease (diabetes).
Type 1 diabetes is an auto¬immune disease that wipes out insulin-producing pancreatic beta cells from the body and raises blood glucose to dangerously high levels. These high levels of Blood sugar level can be even fatal. Patients are being administered insulin and given other medications to maintain blood sugar level. To those who cannot maintain their blood sugar level, they are given beta-cell transplants but to tolerate beta cell transplants; patients have to take immunosuppressive drugs as well.
A report by a research group at Harvard University tells us that they used insulin-producing cells derived from human embryonic stem cells (ESCs) and induced pluripotent stem cells to lower blood glucose levels in mice. Nowadays, many laboratories are getting rapid progress in human stem cell technology to develop those cells that are functionally equivalent to beta-cells and the other pancreatic cell types. Other groups are developing novel biomaterials to encapsulate such cells and protect them against the immune system without the need for immunosuppressant.
Major pharmaceutical companies and life sciences venture capital firms have invested more than $100 million in each of the three most prominent biotechnological industries to bring such treatments into clinical use:
- Cambridge
- Massachusetts–based companies Semma Therapeutics
- Sigilon Therapeutics, and ViaCyte of San Diego
Researchers of UC San Francisco have transformed human stem cells into mature insulin-producing cells for the first time, a breakthrough in the effort to develop a cure for type-1 (T1) Diabetes. Replacing these cells, which are lost in patients with T1 diabetes, has long been a dream of regenerative medicine, but until now scientists had not been able to find out how to produce cells in a lab dish that work as they do in healthy adults.
What is T1 diabetes?
T1 diabetes is an autoimmune disorder that destroys the insulin-producing beta cells of the pancreas, typically in childhood. Without insulin’s ability to regulate glucose levels in the blood, spikes in blood sugar can cause severe organ damage and eventually death. The condition can be managed by taking regular shots of insulin with meals. However, people with type 1 diabetes still often experience serious health consequences like kidney failure, heart disease and stroke. Patients facing life-threatening complications of their condition may be eligible for a pancreas transplant from a deceased donor, but these are rare, and they are supposed to wait a long time.
Researchers have just made a breakthrough that might one day make these technologies obsolete, by transforming human stem cells into functional insulin-producing cells (also known as beta cells) – at least in mice.
“We can now generate insulin-producing cells that look and act a lot like the pancreatic beta cells you and I have in our bodies,” explains one of the team, Matthias Hebrok from the University of California San Francisco (UCSF).
“This is a critical step towards our goal of creating cells that could be transplanted into patients with diabetes.”
Type-1 diabetes is characterized by a loss of insulin due to the immune system destroying cells in the pancreas – hence, type 1 diabetics need to introduce their insulin manually. Although this is a pretty good system, it’s not perfect.
Making insulin-producing cells from stem cells
Diabetes can be cured through an entire pancreas transplant or the transplantation of donor cells that produce insulin, but both of these options are limited because they rely on deceased donors. Scientists had already succeeded in turning stem cells into beta cells, but those cells remained stuck at an early stage in their maturity. That meant they weren’t responsive to blood glucose and weren’t able to secrete insulin in the right way.
Scientists at the University of California San Francisco made a breakthrough in the effort to cure diabetes mellitus type 1.
For the first time, researchers transformed human stem cells into mature insulin-producing cells, which could replace those lost in patients with the autoimmune. There is currently no known way to prevent type-1 (T1) diabetes, which destroys insulin production in the pancreas, limits glucose regulation, and results in high blood sugar levels. The condition can be managed with regular shots of insulin, but people with the disease often experience serious health complications like kidney failure, heart disease, and stroke.
“We can now generate insulin-producing cells that look and act a lot like the pancreatic beta cells you and I have in our bodies,” according to Matthias Hebrok, senior author of a study published last week in the journal Nature Cell Biology.
“This is a critical step toward our goal of creating cells that could be transplanted into patients with diabetes,” Hebrok, director of the UCSF Diabetes Center, said in a statement.
Islets of Langerhans are groupings of cells that contain healthy beta cells, among others. As beta cells develop, they have to separate physically from the pancreas to form these islets.
The team artificially separated the pancreatic stem cells and regrouped them into these islet clusters. When they did this, the cells matured rapidly and become responsive to blood sugar. In fact, the islet clusters developed in ways “never before seen” in a lab. After producing these mature cells, the team transplanted them into mice. Within days, the cells were producing insulin similar to the islets in the mice. While the study has been successful in mice, it still needs to go through more rigorous testing to see if it would work for humans as well. But the research is up-and-coming. “We can now generate insulin-producing cells that look and act a lot like the pancreatic beta cells you and I have in our bodies. This is a critical step towards our goal of creating cells that could be transplanted into patients with diabetes,” He said.
“We’re finally able to move forward on several different fronts that were previously closed to us,” he added. “The possibilities seem endless.”
Basic research keeps elucidating new aspects of beta cells; there seem to be several subtypes, so the gold standard for duplicating the cells is not entirely clear. Today, however, there is “a handful of groups in the world that can generate a cell that looks like a beta cell,” says Hebrok, who currently acts as scientific advisor to Semma and Sigilon, and has previously advised ViaCyte. “Certainly, companies have convinced themselves that what they have achieved is good enough to go into patients.”
The stem cell reprogramming methods that the three companies use to prompt cell differentiation create a mixture of islet cells. Beta cells sit in pancreatic islets of Langerhans alongside other types of endocrine cells. Alpha cells, for example, churn out glucagon, a hormone that stimulates the conversion of glycogen into glucose in the liver and raises blood sugar. Although the companies agree on the positive potential of islet cell mixtures, they take different approaches to developing and differentiating their cells. Semma, which was launched in 2014 to commercialize the Harvard group’s work and counts Novartis among its backers, describes its cells as fully mature, meaning that they are wholly differentiated into beta or other cells before transplantation. “Our cells are virtually indistinguishable from the ones you would isolate from donors,” says Semma chief executive officer BastianoSanna
To get around the donor problem, researchers, including the team at UCSF has been working on nudging stem cells into becoming fully-functional pancreatic beta cells for the last few years. Still, there have been some issues in getting them all the way there.
“The cells we and others were producing were getting stuck at an immature stage where they weren’t able to respond adequately to blood glucose and secrete insulin properly,” Hebrok said.
“It has been a major bottleneck for the field.”
“We’re finally able to move forward on a number of different fronts that were previously closed to us,” Hebrok added. “The possibilities seem endless.”
Regardless of starting cell type, the companies say they are ready to churn out their cells in large numbers. Semma, for example, can make more islet cells in a month than can be isolated from donors in a year in the United States, Sanna says, and the company’s “pristine” cells should perform better than donor islets, which are battered by the aggressive techniques required for their isolation.
As these products, some of which have already entered clinical trials, move toward commercialization, regulatory agencies such as the US Food and Drug Administration (FDA) and the European Medicines Agency have expressed concern about the plasticity of the reprogrammed cells. All three firms subject their cells to rigorous safety testing to ensure that they don’t turn tumorigenic. Before successful trials, companies won’t know the dose of beta cells required for a functional cure, or how long such “cures” will last before needing to be boosted. There’ll be commercial challenges, too: while the companies are investing heavily to develop suitable industrial processes, all acknowledge that no organization has yet manufactured cell therapies in commercial volumes.
Nevertheless, there’s growing confidence throughout the field that these problems will be solved, and soon. “We have the islet cells now,” says Alice Tomei, a biomedical engineer at the University of Miami who directs DRI’s Islet Immuno-engineering Laboratory.
“These stem cell companies are working hard to try to get FDA clearance on the cells.”
Protecting stem cell therapies from the immune system
Whatever the type of cell being used, another major challenge is delivering cells to the patient in a package that guards against immune attack while keeping cells fully functional. Companies are pursuing two main strategies:
- Microencapsulation, where cells are immobilized individually or as small clusters, in tiny blobs of a biocompatible gel.
- Macroencapsulation, in which greater numbers of cells are put into a much larger, implantable device.
ViaCyte, which recently partnered with Johnson & Johnson, launched its first clinical trial in 2014. The trial involved a micro-encapsulation approach that packaged up the company’s partially differentiated, ESC-derived cells into a flat device called the PEC-Encapsulation. About the size of a Band-Aid, the device is implanted under the skin, where the body forms blood vessels around it. “It has a semipermeable membrane that allows the free flow of oxygen, nutrients, and glucose,” says ViaCyte’s chief executive officer, Paul Laikind. “And even proteins like insulin and glucagon can move back and forth across that membrane, but cells cannot.”
The trial showed that the device was safe, well-tolerated, and protected from the adaptive immune system—and that some cells differentiated into working islet cells. But most cells didn’t engraft effectively because a “foreign body response,” a variant of wound healing, clogged the PEC-Encap’s membrane and prevented vascularization. ViaCyte stopped the trial and partnered with W. L. Gore & Associates, the maker of Gore-Tex, to engineer a new membrane. “With this new membrane,” says Laikind, “we’re not eliminating that foreign body response, but we’re overcoming it in such a way that allows vascularization to take place.” The company expects to resume the trial in the second half of this year, provided it receives the green light from the FDA.
Semma is also developing macro¬-encapsulation methods, including a very thin device that in prototype form is about the size of a silver dollar coin. The device is “deceptively simple, but it allows us to put [in] a fully curative dose of islets,” Sanna says.
Semma is also investigating microencapsulation alternatives. At the same time, the company is advancing toward clinical trials using established transplantation techniques to administer donated cadaver cells to high-risk patients who find it particularly difficult to control their blood glucose levels. These cells are infused via the portal vein into the liver, and patients take immunosuppressive drugs to prevent rejection.
Sigilon is working on its microencapsulation technology. Launched in 2016 on the back of work by the labs of Robert Langer and Daniel Anderson at MIT, the company has created 1.5-millimeter gel-based spheres that can hold between 5,000 and 30,000 cells (Nat Med, 22:306–11, 2016). Each sphere is like a balloon, with the outside chemically modified to provide immune-protection, says Sigilon chief executive officer Rogerio Vivaldi. “The inside of the balloon is full of a gel that creates almost a kind of a matrix net where the cells reside.”
In 2018, shortly after partnering with Eli Lilly, Sigilon and collaborators published research showing that islet cells that were encapsulated in gel spheres and transplanted into macaques remained functional for four months. The company has not disclosed a time frame for a type 1 diabetes trial “but we’re moving pretty quickly,” says chief scientific officer David Moller.
Conclusion
To conclude, all three firms hope to extend their work to treat some of the 400 million people worldwide with type 2 diabetes, many of them eventually benefit from insulin injections. The recent endorsements from big Pharmaceutical underline the real progress in beta-cell transplants, says Aaron Kowalski, a molecular geneticist and chief executive officer at JDRF, a foundation based in New York that has funded research at ViaCyte and academic labs whose work has been tapped by Semma and Sigilon. “These companies all realize that if they don’t do it, somebody else will. It’s hard to predict exactly when, but somebody is going to make this work.”
- Published in Corporate News / Blog
Global Stem Cells Group Announces New Business Development Director for Africa and Europe
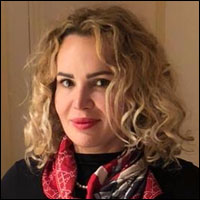
Holya Aziza El Materi Ep Khemiri, M.D.
Global Stem Cells Group announces the appointment of Holya Aziza El Materi Ep Khemiri, M.D. as Vice President Business Development Africa and Europe. Khemiri will oversee development of a new Stem Cell Center in Tunisia.
MIAMI, Nov. 1, 2018—Global Stem Cells Group founder and CEO Benito Novas has named Holya Aziza El Materi Ep Khemiri, M.D. Vice President Business Development for Africa and Europe. The announcement is the latest update on the regenerative medicine organization’s expansion of its clinical presence on the two continents.
Khemiri, who specializes in pharmaceutical and parapharmacy medicines, product manufacturing and medical industry business development, will be in charge of developing and opening a new Stem Cell Center in Tunisia focused on the treatment of patients with degenerative illnesses and orthopedic impairments, and professional athletes with sports injuries.
Stem Cell Center Tunisia will provide regenerative medicine training to African physicians who wish to offer stem cell therapies in their practices.
Since opening the first African Stem Cell Center in Casablanca, Morocco in July, 2017, GSCG has accelerated momentum to bring regenerative medicine, training and education to Africa and Europe through expanded networking opportunities introducing the organization’s authoritative training programs, workshops, seminars, online marketplace for regenerative medicine products, expert instruction and advice on marketing for regenerative practices and other innovative initiatives.
Stem Cell Center Tunisia is part of Global Stem Cells Group’s strategy to facilitate international growth and bring quality-of-life-enhancing regenerative medicine to patients in every corner of the world. The Tunisian center’s medical team will work closely with regional patients, educators and regulators to foster engagement and promote stem cell medicine technology.
ction as a regenerative medicine reference for physicians in North Africa, providing physicians with instruction in stem cell therapies and protocols Those wishing to implement stem cell oversight in their practices will be able to visit the office and collaborate other physicians, participate in educational classes and workshops, and receive the training necessary to start implementing regenerative medicine in their practices and clinics.
Additionally, Stem Cell Center Tunisia will offer physicians a stem cell processing center, GSCG’s complete solution for onsite tissue processing, isolation, culturing and cryopreservation of stem cells. One of the biggest challenges of using traditional stem cell harvesting protocols in medical office settings is obtaining enough stem cells to treat certain degenerative conditions such as autism, lupus. Parkinson’s Disease, Alzheimer’s Disease and others.
The stem cell processing center provides a solution that allows physicians to expand stem cell colonies and obtain a significantly larger number of cells sufficient to treat patients. It also helps with patients needing multiple stem cell treatments by allowing the physician to perform a single extraction of stem cells from fat tissue; the cells harvested can then be saved and expanded, eliminating the need for new cell extraction with each treatment.
According to Novas, Khemiri’s skills and experience make her an ideal candidate to spearhead Stem Cell Center Tunisia’s development and opening.
“Dr. Khemiri brings an array of expertise and knowledge to our expansion efforts in Africa and Europe,” says Novas. She joins Global Stem Cells Group’s management team as a welcome addition at a time when our international expansion endeavors are growing exponentially.”
Khemiri was president of Tunisia’s Society Isotope Radioavtive (SISORA) from 2011 to 2014, where she was involved in manufacturing radioactive tracers to detect cancer. As president of the organization, she helped establish a partnership between SISORA and Belgium-based Radio Pharma Solultions (IBA).
From 2008 to 2011, she served as Research and Development Director of ADWYA, a Tunisia-based company specializing in the production of pharmaceutical products for medical and veterinary use and the country’s first private pharmaceutical laboratory and one of its largest private pharmaceutical companies.
In 2010, she served as creative director of RAD where she focused on formula and scientific studies and registration of medicines, skin care and food supplements with the Tunisian Health Ministry.
Khemari received her medical training at the Catholic University of Louvain in Brussels, Belgium from 1999 to 2006. She received a diploma in Pharmaceutical Sciences from the the Institut Roger Lambion in Brussels and a diploma in Biochemistry, Cosmetology, and Science Perfumery from the University Libre in Brussels.
For more information, visit the Global Stem Cells Group website, email info@stemcellsgroup.com or call 305-560-5337.
About Global Stem Cells Group:
Global Stem Cells Group (GSCG) is a worldwide network that combines seven major medical corporations. Each corporation is focused on furthering scientific and technological advancements in cutting-edge stem cell research, development, treatment, and training. The united efforts of GSCG’s affiliate companies provide medical practitioners with a one-stop epicenter for stem cell solutions that adhere to the highest medical standards.
Global stem cell’s mission is to be the largest recognized stem cell and regenerative medicine network in the world. About Adimarket: Adimarket, Inc., a division of the Global Stem Cells Group, is a one-stop, cost-competitive online marketplace for quality regenerative medicine equipment and supplies for physicians and healthcare professionals.
###
Holya Aziza El Materi Ep Khemiri named Vice President Business Development Africa and Europe.
- Published in Press Releases
Stem Cell Research and Stem Cell Therapy: When can stem cells be used to treat patients?
The difference between stem cell research and therapy is in the scientific evidence that supports therapeutic intervention to be beneficial for the patient.
Stem cells have the remarkable potential to develop into many different types of cells in the body during early life and growth. In addition, in many tissues, stem cells serve as a sort of internal repair system, dividing essentially without limit to replenish other cells as long as the individual is alive. When a stem cell divides, each new cell has the potential either to remain a stem cell or become another type of cell with a more specialized function, such as a muscle cell, a red blood cell, or a brain cell.
Stem cell research on adult stem cells
Stem cells are distinguished from other cell types by two important characteristics. First, they are unspecialized cells capable of renewing themselves through cell division, sometimes after long periods of inactivity. Second, under certain physiologic or experimental conditions, they can be induced to become tissue- or organ-specific cells with special functions. In some organs, such as the gut and bone marrow, stem cells regularly divide to repair and replace worn out or damaged tissues. In other organs, such as the pancreas and the heart, stem cells only divide under special conditions.
Until recently, scientists primarily worked with two kinds of stem cells from animals and humans: embryonic stem cells and non-embryonic “somatic” or “adult” stem cells in stem cell research.
In 2006, researchers made a breakthrough by identifying conditions that would allow some specialized adult cells to be “reprogrammed” genetically to assume a stem cell-like state. This new type of stem cell is called induced pluripotent stem cells (iPSCs).
Stem cells are important for living organisms for many reasons. In the 3- to 5-day-old embryo, called a blastocyst, the inner cells give rise to the entire body of the organism, including all of the many specialized cell types and organs such as the heart, lungs, skin, sperm, eggs and other tissues. In some adult tissues, such as bone marrow, muscle, and brain, discrete populations of adult stem cells generate replacements for cells that are lost through normal wear and tear, injury, or disease.
Stem cell research for treating disease
Given their unique regenerative abilities, stem cells offer new potentials for treating diseases such as diabetes, and heart disease. However, much work remains to be done in the laboratory and the clinic to understand how to use these cells for cell-based therapies to treat disease, which is also referred to as regenerative or reparative medicine.
Laboratory studies of stem cells enable scientists to learn about the cells’ essential properties and what makes them different from specialized cell types. Scientists are already using stem cells in the laboratory to screen new drugs and to develop model systems to study normal growth and identify the causes of birth defects.
Research on stem cells continues to advance knowledge about how an organism develops from a single cell and how healthy cells replace damaged cells in adult organisms. Stem cell research is one of the most fascinating areas of contemporary biology, but, as with many expanding fields of scientific inquiry, research on stem cells raises scientific questions as rapidly as it generates new discoveries.
In 1964, the World Medical Association developed the Declaration of Helsinki as a statement of
ethical principles for medical research involving human subjects. It includes research on identifiable human material and data, last amended in October 2013.
According to the Helsinki Declaration, in the treatment of an individual patient where proven interventions do not exist or other known interventions have been ineffective, the physician, after seeking expert advice, with informed consent from the patient or a legally authorized representative, may use an unproven intervention if in the physician’s judgement it offers hope of saving life, re-establishing health or alleviating suffering.
Intervention should subsequently be made the object of research, designed to evaluate its safety and efficacy. In all cases, new information must be recorded and, where appropriate, made publicly available.
- Published in Corporate News / Blog
Stem Cell Myths, Busted
The term stem cell research gleans different reactions from people, both in the medical community and the wider public. Still an emerging science, stem cell research is shrouded by many myths and misconceptions. Here, we take on some of the most predominant myths to discuss the misconceptions and clarify the facts regarding this fast-growing branch of medicine.
Stem cell myths
Myth #1: Stem cells only come from embryos.
FACT: False. Stem cells exist in all bodies, from embryos to adults.
Embryonic stem cells come from the early embryo, and have the potential to produce all the specialized cells of the body. Because of this, they hold great promise for studying and potentially treating disease and injuries. Tissue or “adult” stem cells are found in the body throughout our lives. These cells maintain and repair many tissues in the body. Examples of these cells include blood stem cells, muscle stem cells, bone marrow stem cells, adipose tissue (fat) stem cells and skin stem cells. Some of these adult stem cells are used in established medical and aesthetic treatments.
Myth #2: Induced pluripotent stem cells (iPSCs) eliminate the need for embryonic cells
FACT: False. Research is needed on all types of cells because it is not clear which cells will be most useful for which types of application. For the foreseeable future, side-by-side research on both embryonic and induced pluripotent stem cells is needed. Global Stem Cell Group’s research and treatment products use no embryonic stem cells.
Myth #3: Stem cell research leads to cloning humans.
FACT: False. Most countries prohibit this type of cloning.
In most countries, even attempting to clone a human being is illegal. Some countries do allow something called “therapeutic cloning” for the purposes of studying a disease. In this procedure, scientists isolate embryonic stem cells from a cloned blastocyst (early stage embryo) but do not transfer the blastocyst into a womb. In therapeutic cloning, the blastocyst is not transferred to a womb. Instead, embryonic stem cells are isolated from the cloned blastocyst. These stem cells are genetically matched to the donor organism for studying genetic disease. For example, stem cells could be generated using the nuclear transfer process described above, with the donor adult cell coming from a patient with diabetes or Alzheimer’s. The stem cells could be studied in the laboratory to help researchers understand what goes wrong in diseases like these.
Therapeutic cloning also could be used to generate cells that are genetically identical to a patient’s. A patient transplanted with these cells would not suffer the problems associated with transplant rejection. To date, no human embryonic stem cell lines have been derived using therapeutic cloning.
Myth #4: Adult stem cells are only found in adults
FACT: False. There are three different types of stem cells: embryonic stem cells, induced pluripotent stem cells and tissue specific stem cells. It’s the tissue stem cells that are often called “adult” stem cells, but these “adult” stem cells are found in people of all ages. (See myth #1).
Stem cell myths: research
Myth #5: Embryonic stem cell research is banned in Europe.
FACT: False. The laws vary across the EU.
EU member states have diverging regulatory positions on human embryonic stem cell research. For instance, in Germany, the use of embryos for research is heavily restricted under the Embryo Protection Act (Embryonenschutzgesetz) of 1991, which makes the derivation
of embryonic stem cell lines a criminal offense. But in the UK, embryonic stem cell research is allowed, subject to licensing from the Human Fertilization and Embryology Authority (HFEA). Click here for country by country overviews for more details. Under the previous two European Framework programs (FP6 and F7), as well as the current program, Horizon 2020, human embryonic stem cell research can be funded, provided that the work is permitted by law in the country where it is to take place.
Myth #6: Stem cell research and treatment is against the law in the US.
FACT: False. The FDA does not regulate the practice of medicine, but rather drugs and medical devices and which of these can be marketed in the US. Under federal law, cultured (grown) stem cell products are considered a drug, but are not illegal. Adult stem cells, however, are not cultured—they exist in our bodies throughout our organs, blood, skin, teeth, fat, bone marrow and other places.
Adult stem cell therapy is currently used in the United States to treat conditions such as leukemia and other illnesses. Bone marrow consists of stem cells which have been transplanted for years in the US.
Global Stem Cells Group offers stem cell treatments in countries where stem cell therapy is approved and regulated with no appreciable difference in safety record.. Stem cell therapy technology is still under review by the FDA.
Stem cell myths: therapies
Myth #7: Bone marrow is the best source of stem cells.
FACT: False. Bone marrow is just one source of stem cells. Bone marrow stem cells have been studied for decades, and have been used to treat certain types of cancer. A great deal of research has been dedicated to understanding this source of stem cells and their potential. Bone marrow contains a number of different kinds of stem cells, one of which is mesenchymal stem cells. However, mesanchymal stem cells can also be found in adipose (fat) tissue at nearly 2000 times the frequency of bone marrow.
Mesenchymal cells have the capability to become different types of tissues (blood vessels, muscle tissue, etc.) and are capable of communicating with other cells. In combination with other proteins, molecules and regenerative cells found in adipose tissue, they also have the ability to reduce inflammation, regenerate damaged tissue, and grow new blood vessels, a process known as angiogenesis. Stem cells from adipose tissue are more accessible and abundant. They can be processed immediately and reintroduced into the body right away.
Myth #8: There is a risk of rejection with stem cell therapy.
FACT: False. When a patient’s stem cells are derived from his or her own body (such as fat tissue), there is no risk of rejection. In fact, studies thus far have indicated no safety issues with fat-derived autologous (from self) stem cells. Since these stem cells come from your own body, the risk of rejection is eliminated.
###
- Published in Corporate News / Blog
How Stem Cell Therapies Can Help Heal Sports Injuries
Continuing our recent discussion of stem cell therapies for sports injuries, the use of mesanchysmal stem cells (MSCs) in orthopedic medicine can help in the repair of damaged tissue by harnessing the healing power of undifferentiated cells that form all other cells in our bodies. The process involves isolating these stem cells from a sample of your blood, bone marrow or adipose tissue (fat cells), and injecting it into the damaged body part to promote healing. Platelet-rich-plasma (PRP), a concentrated suspension of platelets (blood cells that cause clotting of blood) and growth factors, is also used to assist the process of repair.
Below are some examples of injuries and areas of research involving the use of mesenchymal stem cells (MSCs), which are (adult) tissue stem cells that are not only able to produce copies of themselves, but also able to divide and form bone, cartilage, muscle, and adipose (fat) stem cells when cultured under certain conditions:
Cartilage Damage
Cartilage has long been considered as an ideal candidate for cell therapy as it is a relatively simple tissue, composed of one cell type, chondrocytes, and does not have a substantial blood-supply network. Of particular interest to researchers is repair of cartilage tissue in the knee, also called the meniscus of the knee. The meniscus is responsible for distributing the body’s weight at the knee joint when there is movement between the upper and lower leg. Only one third of meniscus cartilage has a blood supply, and as the blood supply allows healing factors and stem cells attached to the blood vessels (called perivascular stem cells) to access the damaged site, the meniscus’s natural lack of blood supply impairs healing of this tissue. Damage to this tissue is common in athletes, and is the target for surgery in 60 percent of patients undergoing knee operations, which usually involves the partial or complete removal of the meniscus, which can lead to long-term cartilage degeneration and osteoarthritis.
Recently, researchers increased their focus on the use of MSCs for treatment of cartilage damage in the knee. Some data from animal models suggest that damaged cartilage undergoes healing more efficiently when MSCs are injected into the injury, and this can be further enhanced if the MSCs are modified to produce growth factors associated with cartilage. It has been shown that once the MSCs are injected into the knee they attach themselves to the site of damage and begin to change into chondrocytes, promoting healing and repair. A small number of completed clinical trials in humans using MSCs to treat cartilage damage have reported some encouraging results, but these studies used very few patients, making it difficult to accurately interpret the results. There are currently a number of ongoing trials using larger groups of patients, and the hope is that these will provide more definite information about the role MSCs play in cartilage repair.
Tendinopathy
Tendinopathy relates to injuries that affect tendons – the long fibrous tissues that connect and transmit force from muscles to bones. Tendons become strained and damaged through repetitive use, making tendinopathy a common injury among athletes. Tendinopathy has been linked to 30 percent of all running-related injuries, and up to 40 percent of tennis players suffer from some form of elbow tendinopathy or “tennis elbow.” Damage occurs to the collagen fibers that make up the tendon, and this damage is repaired by the body through a process of inflammation and production of new fibers that fuse together with the undamaged tissue. However, this natural healing process can take up to a year to resolve, and results in the formation of a scar on the tendon tissue, reducing the tendon’s natural elasticity, decreasing the amount of energy the tissue can store and resulting in a weakening of tendon.
MSCs have the ability to generate cells called tenoblasts that mature into tenocytes. These tenocytes are responsible for producing collagen in tendons. This link between MSCs and collagen is the focus for researchers investigating how stem cells may help treat tendinopathy. Substantial research has been carried out on racehorses as they suffer from high rates of tendinopathy, and the injury is similar to that found in humans. Researchers discovered that by injecting MSCs isolated from an injured horse’s own bone marrow into the damaged tendon, recurrence rates were almost cut in half compared to horses that receive traditional medical management for this type of injury. A later study by the same group showed the MSCs improved repair, resulting in reduced stiffness of the tissue, decreased scarring and better fusion of the new fibers with the existing, undamaged tendon. It is not yet clear if these results are due to MSCs producing new tenocytes or their ability to modulate the environment around the tendinopathy, as described above. These promising results paved the way for the first pilot study in humans.
Bone Repair
Bones are unique in that they have the ability to regenerate throughout life. Upon injury, such as a fracture, a series of events occur to initiate healing of the damaged bone. Initially there is inflammation at the site of injury, and a large number of signals are sent out. These signals attract MSCs, which begin to divide and increase their numbers. The MSCs then change into either chondrocytes, the cells responsible for making a type of cartilage scaffold, or osteoblasts, the cells that deposit the proteins and minerals that comprise the hard bone on to the cartilage. Finally these new structures are altered to restore shape and function to the repaired bone. A number of studies carried out in animals have demonstrated that direct injection or infusing the blood with MSCs can help heal fractures that previously failed to heal naturally. However, as was the case with tendinopathy, it is not yet clear if these external MSCs work by generating more bone-producing cells or through their ability to reduce inflammation and encourage restoration of the blood supply to injured bone, or both.
Brain injury in sports
There is mounting evidence that those taking part in sports where they are exposed to repetitive trauma to the head and brain are at a higher risk of developing neurodegenerative disorders, some of which are targets for stem cell treatments. For example, it has been reported that the rate of these diseases, like Alzheimer Disease, were almost four times higher in professional American football players compared to the general population. While the cause of this disease is not yet clear, it is associated with abnormal accumulation of proteins in neural cells that eventually undergo cell death and patients develop dementia. Researchers have attempted a number of strategies to investigate treatments of this disease in mice, including introducing neural stem cells that could produce healthy neurons. While some of these experiments have demonstrated positive, if limited, effects, to date there are no stem cell treatments available for Alzheimer’s Disease.
Boxers suffering from dementia pugilistica, a disease thought to result from damage to nerve cells, can also demonstrate some symptoms of Parkinson’s Disease (among others). In healthy brains, specialized nerve cells called dopaminergic neurons produce dopamine, a chemical that transmits signals to the part of the brain responsible for movement. The characteristic tremor and rigidity associated with Parkinson’s Disease is due to the loss of these dopaminergic neurons and the resulting loss of dopamine production. Researchers are able to use stem cells to generate dopaminergic neurons in the lab that are used to study the development and pathology of this disease. While a recent study reported that dopaminergic neurons derived from human embryonic stem cells improved some symptoms of the disease in mice and rats, stem cell based treatments are still in the development phase.
- Published in Corporate News / Blog