Adipose Stem Cell Therapies are Revolutionizing Plastic Surgery, and Demand is High
Adipose stem cells (pictured) harvested from body fat. (Photo: Genetic Engineering & Biotechnology News).
The discovery of abundant stem cell populations in body fat tissue changed everything the medical community thought it knew about stem cells overnight. Now, adipose stem cell therapies are driving the plastic and cosmetic surgery industries, and demand among patients keeps rising.
In 2001, researchers and plastic surgeons from the University of Pittsburgh discovered that human fat tissue is a very rich source of mesenchymal stem cells (MSCs), multipotent stromal cells that can differentiate into a variety of cell types. When their findings were published in Tissue Engineering Journal, the discovery stirred quite an epiphany in the medical and scientific community—until then, adult MSCs were predominantly believed to be strictly a bone marrow product.
Adipose tissue offers distinct advantages over bone marrow tissue. Adipose fat is easier to extract than bone marrow, and the stem cell population contained in fat tissue is far more abundant than in bone marrow. One ounce of fat contains 300-500 times as many mesenchymal stem cells as an ounce of bone marrow. And unlike bone marrow, because of autologous adipose tissue’s copious stem cell count, most procedures using them do not require cells to be expanded in a lab, which means that most adipose stem cell therapies can be performed in the same operative procedure. Because bone marrow typically needs to be culture expanded for days in a lab before they can be re-injected back into a patient and adipose cells do not, there are plenty of advantages to adipose stem cell therapies.
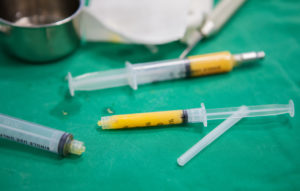
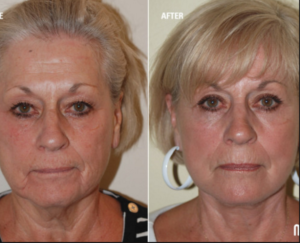
BEFORE AFTER
Cosmetic cell assisted fat transfer
This discovery has created an international demand for stem cell anti-aging therapies, which since these procedures are non-invasive (no surgery involved), make for a faster recovery and significantly less downtime for patients. Many patients and physicians feel that adipose stem cells also create a more natural appearance for recipients than traditional cosmetic surgery procedures. Some cosmetic stem cell physicians have taken it up a notch with cell assisted fat transfer, in which autologous adipose-derived (stromal) stem cells are used in combination with lipoinjection for even softer, more natural results.
Here’s how it works: a stromal vascular fraction (SVF) containing ASCs is freshly isolated from half of the aspirated fat and recombined with the other half. This process converts relatively ASC-poor aspirated fat to ASC-rich fat, reducing the potential for postoperative atrophy of injected fat to a minimal level, which clinical trials have found does not change substantially after two months.
Adipose Tissue as a Regenerative Therapy
While adipose tissue is a definitive source of stem cells, what if you don’t need to isolate or separate the stem cells to benefit from their regenerative powers?
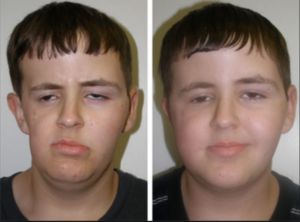
Radiation to treat a tumor severely effected this boy’s facial growth and development, caused significant facial asymmetry and left a large cosmetic defect on the side of his head. Fat grafting surgery restored his appearance to that of any healthy, happy, 14-year-old.
Plastic surgeons have acquired decades of experience in harvesting and refining adipose tissue for treating patients. Thanks to the remarkable level of expertise they have developed with adipose tissue, experts now play a leading role in developing its evolving regenerative applications. Regenerative medicine is changing the landscape of cosmetic and reconstructive surgery, and aesthetic medicine—and it keeps getting better!
###
- Published in Corporate News / Blog
Stem Cell Research Goes Crimson: International Leader in Stem Cell Research Named New Dean of Harvard Medical School
George Q. Daley, MD, PhD, Harvard Medical School’s newly appointed dean, led dozens of international colleagues in developing ethical guidelines for stem cell research. On March 9, 2009, President Barack H. Obama issued Executive Order 13505: Removing Barriers to Responsible Scientific Research involving Human Stem Cells, stating that the Secretary of Health and Human Services, through the Director of the National Institute of Health (NIH), may support and conduct responsible, scientifically worthy human stem cell research, including human embryonic stem cell (hESC) research, to the extent permitted by law. Internal NIH policies and procedures, consistent with Executive Order 13505 and these Guidelines, govern the conduct of intramural NIH stem cell research.
A prominent stem cell researcher has been named the new dean of Harvard Medical School, the university announced August 9th
George Q. Daley, MD, PhD, who led dozens of international colleagues to unite around ethical guidelines for stem cell research, is taking on a new challenge—unifying the powerful hospitals that train Harvard’s medical students.
Daley will assume the position effective Jan. 1, 2017, succeeding Jeffrey Flier, MD, who stepped down July 31st. Barbara McNeil, MD, the founding head of the Department of Health Care Policy at Harvard Medical School, is filling the position in the interim.
The internationally recognized leader in stem cell science and cancer biology and a longtime member of the Harvard Medical School (HMS) faculty whose work includes the fields of basic science and clinical medicine, Daley was the driving force behind creating international guidelines around first, human embryonic stem cell research, and then the clinical application of stem cells, according to Nancy Witty, CEO of the International Society for Stem Cell Research (ISSCR).
Daley, who cofounded the organization, counseled two dozen scientists through the sensitive ethical discussions involved in establishing stem cell research guidelines, utilized additional input from 60 groups around the world to construct the guidelines which were first published by the National Institute of Health in 2009.
“That’s a very difficult task,” Witty said. “It takes a tremendous amount of diplomacy.”
Daley is working to adapt insights in stem cell research to improved therapies for genetic and malignant diseases. Important research contributions from his laboratory at Harvard-affiliated Boston Children’s Hospital include the development of customized stem cells to treat genetic immune deficiency in a mouse model (in collaboration with Rudolf Jaenisch, a Professor of Biology at MIT); the differentiation of germ cells from embryonic stem cells (cited as a “Top Ten Breakthrough” by Science magazine in 2003), and the generation of disease-specific pluripotent stem cells by direct reprogramming of human fibroblasts (cited in the “Breakthrough of the Year” issue of Science magazine in 2008).
As a graduate student working with Nobel laureate Dr. David Baltimore, Daley demonstrated that the BCR/ABL oncogene induces chronic myeloid leukemia (CML) in a mouse model, which validated BCR/ABL as a target for drug blockade and encouraged the development of imatinib (GleevecTM; Novartis), a revolutionary magic-bullet chemotherapy that induces remissions in virtually every CML patient. Dr. Daley’s recent studies have clarified mechanisms of Gleevec resistance and informed novel combination chemotherapeutic regimens.
Daley has spent his entire career in Cambridge and Boston, earning a medical degree from Harvard and a PhD in biology from MIT. As Dean of Harvard Med School, Daley’s achievements in stem cell research is expected to shine a distinguished light on the stem cell industry.
Although the position of dean of Harvard Med may be one of the most prominent roles in medicine, the position is not as powerful it might seem: Harvard Med does not directly oversee any hospitals. Instead it relies on 15 affiliated hospitals and clinical sites, which have historically operated as separate, competitive bailiwicks, to train its students and postdoctoral fellows, and support its researchers. Only 151 of the nearly 12,000 people who call themselves Harvard Medical faculty actually work directly for Harvard in its 10 basic science departments.
Daley sees his new position as a congregator who “builds bridges among the institutions” —heavyweight research institutions such as Brigham and Women’s, Massachusetts General, and Boston Children’s hospitals. Persuasiveness, rather than power, is all that Daley says is needed to achieve an alliance.
Daley’s predecessor, Flier, says he spent a full 30 percent to 40 percent of his time as dean trying to build relationships with and coordinate Harvard’s affiliated hospitals and clinics, a challenge Daley says he’s up to. He has a head start in building those relationships through the many positions he has held around Boston’s biomedical community, including chief resident at Mass. General
“My vision is one of increasing connectivity across the community,” Daley says.
Currently a professor of biological chemistry and molecular pharmacology at Harvard Medical School and director of the stem cell transplantation program at Boston Children’s and Dana-Farber Cancer Institute, Daley sees areas of common interest, such as immuno-oncology, which harnesses the body’s own immune system against cancer cells, where the hospitals can work more closely together.
Described by colleagues as a natural leader, Daley recently led an effort to coordinate big-name scientists across several institutions on a collaborative grant to compare two types of stem cells—just one example of how he earned a reputation; he knows how to get different groups talking together in a constructive way.
He also says he wants Harvard Medical’s faculty, students, and staff to reflect the global community the school intends to serve, and that he promotes diversity in hiring for his 30-person lab.
Daley has a keen interest in sickle-cell anemia, which affects people of African descent, including African Americans, and he believes the federal government should invest in a moonshot effort to cure the disease.
Daley plans to continue teaching molecular medicine at Harvard Med after assuming his position as dean. He also plans to spend one day per week in his lab researching blood stem cells.
“It’s important for a dean to remain relevant by continuing to publish papers,” Daley says. “Plus, I just love science.”
Among his priorities is raising money. Despite its worldwide reputation, and its relative influence when it comes to landing federal grants, Harvard Med has seen annual deficits of between $31 million and $45 million for three consecutive years. Suggestions are being made for Harvard to rename its medical school in return for a billion-dollar donation. Daley only says that the idea would be worth considering down the road.
With opportunities for federal grants in decline, Daley says he sees an opportunity to bring in money from corporate partnerships.
For the stem cell research and medical community, Daley’s appointment as dean of Harvard Med is a fitting step toward validating regenerative medicine’s place as an authoritative leader in the future of medicine—one that’s been a long time coming.
Learn more about Dr. Daley here.
###
- Published in Corporate News / Blog
Texas Man Becomes First Adult in the U.S. to Receive Updated Stem Cell Transplant to Treat Leukemia
Chuck Dandridge, a Mansfield, Texas resident, became the first adult in the U.S. to receive a newly modified stem cell transplant that uses genetically engineered blood cells from a family member. The milestone was announced by researchers at UT Southwestern Medical Center’s Harold C. Simmons Comprehensive Cancer Center in Dallas, where the procedure was performed.
Dandridge’s medical journey began in 2013, with a routine doctor’s visit to check his cholesterol levels; lab tests revealed low blood counts and further testing confirmed Dandridge’s diagnosis of myelodysplastic syndrome, also called pre-leukemia or MDS. By 2014, the leukemia had progressed to acute myeloid leukemia (AML), which, according to the National Cancer Institute, affects more than 20,000 Americans annually.
Dandridge was referred to UT Southwestern’s Simmons Cancer Center, where his leukemia was tested for genetic mutations.
“We wanted to know whether he had specific mutations in his cancer cells,” says
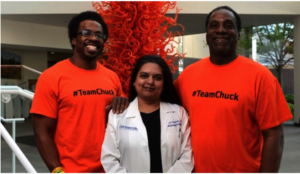
Jon Dandridge, Madhuri Vusirikala, M.D., and Chuck Dandridge at the Simmons Cancer Center. Photo: UT Southwestern Medical Center.
“We found a mutation called IDH 2, which causes the body to produce an abnormal protein that promotes excessive cell growth. If you can target that mutation and stop the abnormal protein from being produced, then cells start behaving normally.”
Dandridge enrolled in a UT Southwestern clinical trial for a therapy called AG-221. He took four pills each morning for the next eight months. During that time, Dandridge saw marked improvement although he did not go into complete remission, according to Vusirikala.
That success made him eligible for a potentially curative stem cell transplant. But finding a donor proved challenging.
“The best chance of finding a full match is usually a full sibling; however, Chuck has no full siblings,” Vusirikala says. Additionally, Dandridge is African American, and minorities are under-represented in the National Marrow Donor Registry—about 70 percent of registry donors are Caucasian. The search for an unrelated donor was unsuccessful.
Vusirikala says that he knew Dandridge’s daughter and his son would be at least a half match. Since using a same-sex donor is preferred, as it reduces the risk of complications, his son Jon, 31, emerged as the best choice. But the risk of graft-versus-host-disease (GvHD) following a transplant using a half-match is very high, so they needed a better way to deal with the GvHD risk.
Once again, Mr. Dandridge volunteered for a cutting-edge clinical trial, known as BP-001, which processed the stem cells used in the transplant to reduce the risk of rejection and engineered blood cells that can be targeted if GvHD develops after the transplant.
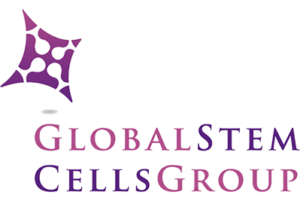
These engineered T cells are modified to include a suicide gene with the help of a retrovirus. If the patient develops GvHD after transplant, the side-effect can be treated by giving a drug called rimiducid to activate the suicide gene and cause the activated GvHD-causing cells to be eliminated. The stem cells given for the transplant were also processed prior to giving them back to Dandridge to reduce the risk of graft rejection as well as GvHD.
The genetically engineered blood cells were transplanted from Dandrige’s son, Jon, 31, to the father in three, two-hour infusions at William P. Clements Jr. University Hospital in July, 2015, and today the elder Mr. Dandridge’s leukemia is in remission. His immune system is recovering, and the former Norman, Oklahoma YMCA CEO is now mentoring first-time CEOs for the YMCA.
###
- Published in Corporate News / Blog
Insulin-producing Stem Cells Grown in the Lab Mark a New Era in Stem Cell Therapies for Diabetes
A new discovery by researchers on how to activate lab-grown beta cells to mature into functioning cells that produce and release insulin in response to glucose take a significant step toward a cell therapy treatment for diabetes.
Difficulties in manipulating beta cells derived from human stem cells to mature beyond the precursor stage into fully functioning insulin releasers has been an on-going challenge for researchers..
However, researchers from the Salk Institute for Biological Studies and a team of researchers have achieved this goal with lab-grown beta cells by activating a protein called estrogen-related receptor γ (ERRγ). Their study findings were recently published in the journal Cell Metabolism.
Self-renewing capacity of human pluripotent stem cells (hPSCs)
Ronald Evans, senior author of the study, titled, “ERRγ Is required for the Metabolic Maturation of Therapeutically Functional Glucose-Responsive β Cells,” says the self-renewing capacity of human pluripotent stem cells (hPSCs) and their ability to differentiate into most cell types—from neurons to skin cells, to muscles cells and insulin-producing pancreatic beta cells—has inspired many research teams to find ways to make glucose-responsive beta cells in the lab.
Evans and his research team discovered the answer to the insulin-releasing cell conundrum, and summed it up thusly:
“In a dish, with this one switch, it’s possible to produce a functional human beta cell that’s responding almost as well as the natural thing.”
Evans, a molecular biologist at the Salk Institute, says that to create the different types of cells in the lab, researchers coax the pluripotent stem cells (hPSCs) down the various branching paths that fetal cells normally travel in order to differentiate into the various cell types. However, he explains there are many developmental points in this process, and in the case of lab-grown pancreatic beta cells, research kept getting stuck at an early stage.
Adult beta cells have more ERRγ protein for a very energy-intensive process
In order to determine what might trigger the next step in getting the cells to mature, the researchers compared transcriptomes of adult and fetal beta cells. The transcriptome contains, among other things, the full catalog of molecules that switch genes on and off in the genome, which led them to discover that the nuclear receptor protein ERRγ was more abundant in adult beta cells. The team was already familiar with the protein’s role in muscle cells and had studied its ability to enhance endurance running.
Evans says that in muscles, protein promotes greater growth of mitochondria—the power generators inside cells that accelerate the burning of sugars and fats to make energy.
“It was a little bit of a surprise to see that beta cells produce a high level of this regulator,” Evans says. “But beta cells have to release massive amounts of insulin quickly to control sugar levels. It’s a very energy-intensive process.”
The research team then decided to run some tests to look more closely at what role ERRγ might play in insulin-producing beta cells.
A new era in creating functional, insulin-producing beta cells
After they genetically engineering a deficiency of ERRy in mice, the researchers found the animals’ beta cells did not produce insulin in response to spikes in blood sugar.
Next they tried to get beta cells made from hPSCs to produce more ERRγ, and it worked! The cells in culture began to respond to glucose and release insulin.
Finally, the team transplanted the lab-grown insulin-producing beta cells into diabetic mice and found that from day one, the cells produced insulin in response to glucose spikes in the animals’ blood.
Evans and the research team were justifiably excited by the results. It appears that just switching on the ERRγ protein is sufficient to get the lab-grown beta cells to mature and produce insulin in response to glucose – both in cultures and in live animals.
Speculating on the implications of their findings, Evans suggests that when a fetus is developing, because it gets a steady supply of glucose from the mother, it does not need to produce insulin to regulate its blood sugar, so the switch is inactive. But, when the baby is born and takes its first breath and takes in oxygen, this activates the switch.
Previous lab attempts to produce beta cells got stuck at the fetal stage. The Salk Institute researchers discovered how to take it to the adult stage, using the same protein that is switched on in nature.
“I believe this work transitions us to a new era in creating functional beta cells at will,” Evans says.
He and his research team now plan to examine how the switch might work in more complex models of diabetes treatments.
The Salk Institute study proceeds another study Medical News Today in which researchers generated mini-stomachs that produce insulin when transplanted into mice.
###
- Published in Corporate News / Blog
Amazing Stem Cell Research Breakthroughs You Never Heard of
Scientists have been studying stem cells for decades, and many of their findings, all pretty remarkable, aren’t widely circulated. Periodically, we will share one of these stem cell research breakthroughs here on this blog.
Summary: The skin renews, heals wounds, and regenerates the hair that covers it thanks to a small group of stem cells. These cells continually produce new ones, which appear on the skin surface after a few days. A 2008, released online July 28, 2016, has identified two proteins that are fundamental to conserve skin stem cells, and shows that without these proteins these cells are lost. Researchers find that these proteins, Dnmt3a and Dnmt3b, are altered similarly to tumor cells found in leukemia, lung cancer and colon cancer, which may help researchers discover if the proteins contribute to tumor development.
Amazing stem cell breakthroughs
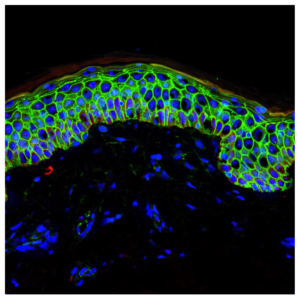
Section of the epidermis showing all its layers, with cell borders in green and cell nuclei in blue (photo: Melissa Mangione)
Researchers identify two proteins— Dnmt3a and Dnmt3b—fundamental to conserving skin stem cells.
The study examines the continuous regeneration of the skin and hair that covers it, thanks to a small group of stem cells. Study researchers identified two proteins— Dnmt3a and Dnmt3b—that are fundamental to conserving skin stem cells. “Without these proteins, skin stem cells are not activated and the stem cells collapse and disappear from the tissue,” according Benitah, head of the Stem Cells and Cancer lab at IRB Barcelona.
Lorenzo Rinaldi, a la Caixa PhD student and first author of the study, identified all the regions of the genome that harbors these proteins. Rinaldi has observed that these two proteins exert their activity on gene enhancers and super-enhancers. Researchers were surprised to see that the two proteins, which had previously been associated with gene repression through DNA methylation, are activated in the most transcriptionally active regions of stem cells.
Researchers observe Dnmt3a and Dnmt3b at the genomic level for the first time
“We had never observed this activity because we were unable to study the global distribution
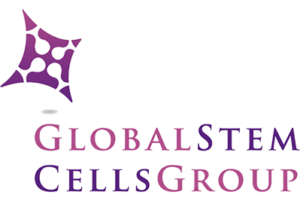
Of the 12,000 gene enhancers in the genome, about 300 are super-enhancers related to stem cells. The two proteins exert their function in these regions in order to trigger the approx. 1,000 genes required for the self-renewing capacity of skin stem cells. By methylating the super-enhancer, these proteins trigger the first step of the machinery that leads to the amplified expression of these essential genes for the stem cell.
Link to cancer
Among the various features related to tumor cells are three components:
• these cells show altered DNA methylation.
• gene enhancers, in addition to the bodies of the genes themselves, are highly mutated. These observations have been made possible thanks to mass sequencing of tumor cell genomes.
• these two proteins, Dnmt3a and Dnmt3b, are altered in many types of tumors, such as those encountered in leukemia, lung cancer and colon cancer.
Each of these three components is associated with the development of various kinds of cancer. Given that these proteins activate gene expression enhancers through DNA methylation, researchers believe that further studies of them in cancer cells would be helpful in determining whether they participate in tumor development.
The study was funded by the Spanish Ministry of Economy and Competitiveness and ERDFs. Benitah’s lab is also supported by The European Council for Research (ERC), the Worldwide Cancer Research Foundation, the Fundació Marató de TV3, the Fundación Vencer el Cáncer, the Fundación Botín and the Government of Catalonia.
###
- Published in Corporate News / Blog
Regenerating and Restoring Brain Cells in the Aged With Donor Neural Stem Cells
The human brain, as it turns out, is far more malleable than we once thought. Even adult brains. But they are subject to age-related diseases and disorders, such as dementia and diminished cognitive function.
There is hope that medical science may be able to replace brain cells and restore memory in aging patients thanks to new discoveries in neural stem cell techniques. Researchers at the Texas A&M Health Science Center College of Medicine recently published new findings in the journal Stem Cells Translational Medicine that suggests a new technique for preparing donor neural stem cells and grafting them into an aged brain can regenerate tissue that has succumbed to structural, chemical, and functional changes, as well as a host of neurocognitive changes that can be attributed to aging.
The study, titled “Grafted Subventricular Zone Neural Stem Cells Display Robust Engraftment and Similar Differentiation Properties and Form New Neurogenic Niches in the Young and Aged Hippocampus,” was led by Ashok K. Shetty, Ph.D., a professor in the Department of Molecular and Cellular Medicine. associate director of the Institute for Regenerative Medicine, and research career scientist at the Central Texas Veterans Health Care System.
Shetty and his team at Texas A&M focus on the aged hippocampus, which plays an important role in making new memories and connecting them to emotions. They took healthy donor neural stem cells and implanted them into the hippocampus of an animal model, essentially enabling them to regenerate tissue.
The hippocampus in the aging brain
“We chose the hippocampus because it’s so important in learning, memory and mood function,” Shetty said. “We’re interested in understanding aging in the brain, especially in the hippocampus, which seems particularly vulnerable to age-related changes.”
The volume of this part of the brain seems to decrease during the aging process, and this decrease may be related to age-related decline in neurogenesis (production of new neurons) and the memory deficits some people experience as they grow older.
The aged hippocampus also exhibits signs of age-related degenerative changes in the brain, such chronic low-grade inflammation and increased reactive oxygen species.
Bharathi Hattiangady, assistant professor at the Texas A&M College of Medicine and co-first author of the study said his team was excited to discover that the aged hippocampus can accept grafted neural stem cells as well as the young hippocampus does, a discovery that has significant implications for treating age-related neurodegenerative disorders.
“It’s interesting that even neural stem cell niches can be formed in the aged hippocampus,” Hattiangady says.
Shetty’s previous research focused on the benefits of resveratrol (an antioxidant that is famously found in red wine and the skin of red grapes, as well as in peanuts and some berries) to the hippocampus. Although the results indicated important benefits for preventing memory loss in aging brains, his newest work demonstrates a way to affect the function of the hippocampus more directly.
Neural stem cell grafting
In this new study, the team found that the neural stem cells engrafted well onto the hippocampus in the young animal models (which was expected) as well as the older ones that would be, in human terms, about 70 years old. Not only did these implanted cells survive, they divided several times to make new cells.
“They had at least three divisions after transplantation,” Shetty said. “So the total yield of graft-derived neurons and glia (a type of brain cell that supports neurons) were much higher than the number of implanted cells, and we found that in both the young and aged hippocampus, without much difference between the two.”
In both old and young brains, a small percentage of the grafted cells retained their stemness feature—an essential characteristic of a stem cell that distinguishes it from ordinary cells—and continuously produced new neurons. This is called creating a new ‘niche’ of neural stem cells, and these niches seemed to be functioning well. They were still producing new neurons at least three months after implantation, and these neurons are capable of migrating to different parts of the brain.
Past efforts to rejuvenate brains using fetal neurons in this way weren’t nearly as successful. Immature cells, such as neural stem cells, seem to do a better job because they can tolerate the hypoxia (lack of oxygen) and trauma of the brain grafting procedure better than post-mitotic or relatively mature neurons. When researchers tried in the past to implant these partially differentiated cells into the aged hippocampus, they didn’t do nearly as well. The research team used a new technique of preparing the donor neural stem cells, which Shetty says is why this result has never been seen before.
Brain marrow
The researchers used donor cells from the sub-ventricular zone of the brain, an area called the “brain marrow,” because it is analogous to bone marrow in that it holds a number of neural stem cells that persist throughout life. These neural stem cells continuously produce new neurons that migrate to the olfactory system. They also respond to injury signals in conditions such as stroke and traumatic brain injury and replace some of the lost cerebral cortical neurons.
Induced pluripotent cells from skin
Even a small stem cell sample is good enough to expand in culture, so the procedure isn’t terribly invasive. However, in the future, a single skin cell might suffice, as similar neural stem cells can be obtained in large numbers from skin. In fact, it is well known in medical science that a number of cells in the body—including skin cells—can be modified in such a way to create induced pluripotent stem cells.
With these cells, scientists can do any number of things, such as making neural stem cells that will make both more of themselves, and make new neurons. It’s not necessary to get the cells from the brain, just take a skin biopsy and push them into neural stem cells, according to Shetty.
Although the way the grafted cells thrived is promising, there is still a good deal of work to be done to determine if the extra grey matter actually improves cognition.
“Next, we want to test what impact, if any, the implanted cells have on behavior and determine if implanting neural stem cells can actually reverse age-related learning and memory deficits,” Shetty said. “That’s an area that we’d like to study in the future.
“I’m always interested in ways to rejuvenate the aged brain to promote successful aging, which we see when elderly persons exhibit normal cognitive function and the ability to make memories.”
###
- Published in Corporate News / Blog
Stem Cell “Tattoo” Technology Allows Researchers to Track Cell Implants Non-invasively
Researchers at the University of Toronto have developed a tracer ink—a “stem cell tattoo”—that provides the ability to monitor stem cells in unprecedented detail after they’re injected.
The research findings, titled “Bifunctional Magnetic Silica Nanoparticles for Highly Efficient Human Stem Cell Labeling,” was published in June in the Journal of Magnetic Resonance Imaging. Already emerging as an ideal probe for noninvasive cell tracking, the technology has the potential to revolutionize stem cell research by arming scientists with the ability to visually follow the pathways and effectiveness of stem cell therapies in the body, in real time.
“Tattoo” tracer can help further development of stem cell therapies
University of Toronto biomedical engineering professor Hai-Ling Margaret Cheng, a biomedical engineer who specializes in medical imaging, says the new technology allows researchers to actually see and track stem cells after they’re injected. Cheng hopes the technique will help expedite the development and use of stem cell therapies.
Working with colleague Xiao-an Zhang, an assistant professor of chemistry at the University of Toronto, Scarborough, Cheng developed a singular chemical compound known as a contrast agent that acts as a tracer. Composed of manganese, an element that naturally occurs in the body, this tracer compound, called MnAMP, bathes stem cells in a green solution, rendering them traceable inside the body under MRI.
Stem cell tracer ink allows long term cell tracking
The contrast agent “ink” first enters a stem cell by penetrating its membrane. Once inside, it stimulates a chemical reaction that prevents it from seeping out of the cell the same way it entered. Previous versions of contrast agents easily escaped cells. By establishing a way to contain the ink within the cell’s walls, the research team achieved the ability to track the cells long term once they are inside the body.
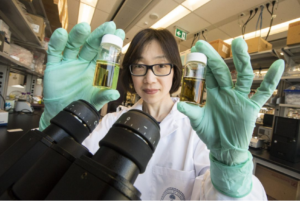
University of Toronto professor Margaret Cheng holds samples of a chemical compound that will create a new way to visualize stem cells inside the body. (Photo: Bernard Weil, Toronto Star)
The thick substance coats the esophagus and other areas of the body with an illuminating compound, making them visible in an x-ray or CT scan. But the barium solution is eliminated from the body within 2 – 3 days or less. Before the stem cell tattoo tracer ink was developed, surgery was the only option for scientists to get a literal glance of a cells’ destiny after it was injected into the body. Now, researchers can track the results in real time, without resorting to any invasive procedures.
“Before, we could not visually track the cells once they were introduced into the body,” Cheng says. “Now we have the ability to view cells in a non-invasive manner using MRI, and monitor them for potentially a very long time.”
Cell tracer technology still in developmental stage
Currently the tracer ink technology is still in the early development phase and requires more animal testing. Cheng is hopeful it can proceed to human clinical trials in about 10 years. While Cheng has already proven that tattooing an animal’s embryonic stem cell doesn’t affect its ability to transform into a functional heart cell, rat, or even a pig (which better represents a human’s size), larger models are up for evaluation next.
In those test cases, researchers will cut off and reduce blood flow in the animals to mimic the effects of damage caused by a human heart attack. Cardiac stem cells pre-tagged with Cheng’s ink tracer technology will then be injected into the damaged tissue. Using MRI to monitor the luminous inked stem cells in action, researchers can non-invasively follow where in the body they’re traveling and more easily determine if the new cells are responsible for restoring normal heart rhythm.
Before it can be tested in humans, the chemical tracer will also have to pass rigorous toxicology tests to ensure its safety.
###
- Published in Corporate News / Blog
Stem Cell-stimulating Fillings Help Regenerate Teeth Damaged by Disease, Decay
Researchers from Harvard University and the University of Nottingham have developed a new filling that stimulates stem cells in dental pulp to regenerate and even regrow teeth damaged by disease and decay. According to Newsweek Magazine, the discovery earned a prize from the Royal Society of Chemistry after judges described it as a “new paradigm for dental treatments.”
The treatment is believed to potentially eliminate the need for root canals.
Filling materials stimulate stem cells to encourage dentin growth
The filling works by stimulating the body’s natural store of stem cells to encourage the growth of dentin—the bony material that makes up the majority of the tooth—allowing patients to effectively regrow teeth that are damaged through dental disease. The filling’s synthetic biomaterials are used similarly to dental fillings, placed in direct contact with pulp tissue in the damaged tooth. This stimulates the tissue’s native stem cell population to repair and regenerate pulp tissue and the surrounding dentin.
The discovery is a significant step forward from current methods to treat cavities, which involve drilling out decay and putting in a filling made of gold; porcelain; silver amalgam (which consists of mercury mixed with silver, tin, zinc, and copper); or tooth-colored plastic or composite resin. When these fillings fail to halt the tooth’s decay, a root canal is needed to remove the pulp of the tooth, damaging it even further.
Alternative to traditional fillings in teeth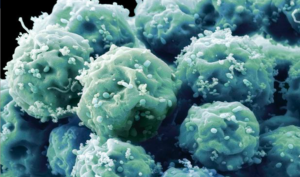
Researchers hope to develop the technique with industry partners in order to make it available for dental patients as an alternative to traditional fillings. Marie Curie research fellow Adam Celiz says that existing dental fillings are toxic to cells and are therefore incompatible with pulp tissue inside the tooth.
“In cases of dental pulp disease and injury, a root canal is typically performed to remove the infected tissues,” Celiz says.
The promise of using therapeutic biomaterials to bring stem cell medicine to restorative dentistry could significantly impact millions of dental patients each year. In fact, the approach is so promising it won second prize in the materials category of the Royal Society of Chemistry’s Emerging Technology Competition for 2016.
Competition entries were judged on the degree of innovation of the technology, its potential impact, and the quality of the science behind it. Increasing innovation in the chemical sciences is a key element of the Royal Society of Chemistry’s industry strategy.
Effective and practical approach to regenerating teeth
The stem cell stimulating filling promises to change the future of dentistry, according to David Mooney, Pinkas Family Professor of Bioengineering at the John Paulson School of Engineering and Applied Sciences at Harvard and the Wyss Institute for Biologically Inspired Engineering.
“’These materials may provide an effective and practical approach to allow a patient to regenerate components of their own teeth,’ Pinkas says.
Stem cells can induce regenerative, self-healing qualities in any tissue found in the body and can, as a result, provide unlimited potential for medical applications. Current studies are underway worldwide to learn how stem cells may be used to prevent or cure diseases and injuries such as Parkinson’s disease, type 1 diabetes, heart disease, spinal cord injury, muscular dystrophy, Alzheimer’s disease, strokes, burns, osteoarthritis, vision and hearing loss, and more. Stem cells may also be used to replace or repair tissue damaged by disease or injury.
###
- Published in Corporate News / Blog
New Guidelines for Stem Cell Research and Therapies Aim to Protect Patients from Charlatan Quackery
Stem cell research has never been more advanced, and as a result many different types of treatments are currently offered on the market. Unfortunate
ly, some providers are practicing quackery in stem cell therapies, and an abundance of well-intentioned scientific and medical personnel are prematurely publicizing their work. These providers and publishers have cast an unfair shadow of mistrust on this very important branch of medical research and potential treatments.
On the other hand, the contributions of professional medical and stem cell societies and other organizations require self-regulation through accreditation and certification, development of standards, and creation of a platform for collaboration among stakeholders.
Professional Guidelines for responsible Stem Cell Research
International Society for Stem Cell Research (ISSCR) is the largest professional organization of stem cell scientists. In 2007, ISSCR impaneled a broad international taskforce to develop a set of professional guidelines for responsible translational stem cell research. Their principles include high standards of preclinical evidence, peer review, scrupulous review of clinical protocol by an Institutional Review Board (IRB), rigorous informed consent, and publication of results whether positive or negative.
The general scientific consensus is that most stem cell therapies are not ready for marketing or commercialization. But the industries that are providing these treatments are increasingly sophisticated and organized, and are challenging established regulatory frameworks.
The International Society for Cellular Therapy (ISCT) has an interest in the promotion of stem cell research and development, but it also is interested in a broader range of cell-based interventions such as immune cell interventions, reproductive medicine, and gene therapy. The ISCT taskforce has working groups on definitions, scientific evidence and biological rationale, laboratory cell processing, clinical practice, regulation, commercial implications, communications, and policy.
Develop terminology, define levels of scientific evidence in new guidelines for stem cell research
The key goals are to develop an appropriate terminology, define the levels of scientific evidence needed to justify routine use or commercialization of a stem cell therapy, address questions of “experimental” and “innovative” use, and understand the global regulatory landscape in order to identify gaps and contradictions.
The ISSCR published revised guidelines for research and clinical translation involving stem cells on May 12, 2016. These new guidelines update and combine guidelines on stem cell research and clinical translation previously issued in 2006 and 2008 Jonathan Kimmelman, Associate Professor of Biomedical Ethics at McGill University, chaired the ISSCR Guidelines Update Task Force. The task force was made up of 25 experts in basic research, clinical research, and bioethics, and received feedback from 85 external individuals and organizations.
2016 guidelines: covering new ground in stem cell research
The 2016 guidelines cover new ground in areas such as gene editing and induced pluripotent stem cells. They introduce a new focus on the communication of results. The task force recognizes that results and potential applications can be exaggerated, leading to distorted understandings of research outcomes in the scientific community, popular press, and among potential patients. The “14-day rule” limiting experimentation on human embryos or embryo-like structures is upheld in these guidelines, although one task-force member has suggested that this may soon be open to revision.
In May, 2016 ISSCR released the following list of all of the new topics addressed in the revised guidelines as part of the announcement of its report:
- Define an Embryo Research Oversight (EMRO) process to encompass both human embryonic stem cell research and human embryo research that may not explicitly pertain to stem cells or generating new stem cell lines;
- Exclude the generation of induced pluripotent stem cells (iPS cells) from specific stem cell research oversight, and instead call on the existing human subjects review processes to oversee donor cell recruitment (iPS cells behave like embryonic stem cells but are derived by reprogramming more differentiated tissue cells);
- Support laboratory-based research that entails gene editing of the nuclear genomes of human sperm, egg, or embryos, when performed under rigorous review, but hold that any attempt to apply this clinically would be premature and should be prohibited at this time;
- Define principles for evaluating both basic and clinically applied research on mitochondrial replacement therapy, in concordance with recent deliberations in the U.K., U.S., and elsewhere;
- Determine that where there is no undue financial inducement to participate, it may be acceptable to compensate women who donate eggs for research;
- Recognize that the development of increasingly complex in vitro models of early stages of human development should undergo specialized review;
- Highlight opportunities to strengthen preclinical studies in stem cell research, including reproducibility and stringent standards for experimental design;
- Call for robust standards for preclinical and clinical research evidence as clinical trials progress and rigorous evaluation for safety and efficacy before marketing approval;
- Address the valuable contributions made by patients or patient groups to support clinical research and a framework to ensure this is achieved without compromising the integrity of the research;
- Highlight the responsibility of all groups communicating stem cell science and medicine—scientists, clinicians, industry, science communicators, and media—to present accurate, balanced reports of progress and setbacks.
The good news is that stem cell research is evolving into a highly respected and in-demand branch of healing that many consider to be the future of medicine. Since pluripotent stem cells have the ability to differentiate into any type of cell, they are used in the development of medical treatments for a wide range of conditions including physical trauma, degenerative conditions, and genetic diseases (in combination with gene therapy). Further treatments using stem cells are being developed due to stem cells’ ability to repair extensive tissue damage.
Great levels of success and potential have been achieved from research using adult stem cells. In early 2009, the FDA approved the first human clinical trials using embryonic stem cells. Embryonic stem cells are pluripotent, which means they can become any cell type of the body, with the exception of placental cells. More and more is being discovered about the plasticity of adult stem cells, increasing the potential number of cell types an adult stem cell can become.
###
- Published in Corporate News / Blog
Researchers Move Closer to Lung Stem Cell Therapies to Treat Chronic Lung Diseases
Chronic lung diseases are the third leading causes of death in the U.S. Chronic lung diseases include a collection of illnesses that cause airflow blockage and breathing-related issues, including primarily chronic obstructive pulmonary disease (COPD), bronchitis, emphysema and asthma. Lung disease involves changes in cells within the lungs, and while research on lung stem cell therapies may not only shed light on their causes, it may provide the groundwork for future treatments.
Stem cells in the lung
Human lungs are hard working organs. In an average lifetime, human lungs take 20-40 million breaths and experience a daily airflow of between 1,850 and 2,640 gallons. Human lungs are made up of two distinct regions:
- The conducting airway tubes, including the trachea, bronchi, and bronchioles.
- The gas exchange regions, or alveolar spaces.
Medical researchers have discovered that these regions each contain unique types of stem cells and progenitor cells. In normal lungs, an abundance of progenitor cells is present in each region, which divide to replace old or damaged lung cells to keep the lungs healthy. The progenitor cells include tracheal basal cells, bronchiolar secretory cells (known as club cells), and alveolar type 2 cells. Progenitor cell division is believed to be sufficient to renew the lung’s structure throughout normal adult life.
Stem cells are far less abundant than progenitors, but are found in both embryonic and adult lungs. Some stem cells assist in initial lung development, while others help repair and regenerate the lung throughout one’s lifetime. Problematic stem cells may actually contribute to lung diseases. In mouse lungs, certain rare stem cells have been located in the conducting airway tubes after to severe injury—for example, flu infection. These rare cells can divide and produce new cells that contribute to both the airway and gas exchange regions. These cells have also been grown in vitro and used as a proof-of-concept treatment in injured mouse lungs.
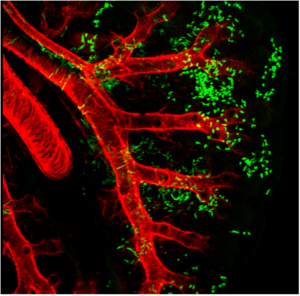
Wnt2+ CPPs (green cells) populate multiple cell lineages in the developing lung including airway and vascular smooth muscle. The smooth muscle of the branching airways and large blood vessels are stained in red.
Adult mesenchymal stem cells (hMSCs)
Adult human mesenchymal stem cells (hMSCs) are the focus of a number of clinical applications. The advantage of hMSCs is that they are immuno-modulatory— capable of modifying or regulating one or more immune functions—and versatile due to the anti-inflammatory and regenerative bioactive molecules they secrete.
hMSCs have the potential to orchestrate reparative processes in diseased or injured tissues. Much of the diversity and uniqueness of hMSCs is defined by their response to the environment of injured tissue. hMSCs are sensitive to their site-specific microenvironment, and scientists anticipate that these cells will deliver the bioactive agents in a site-specific manner quite different from the way pharmaceutical drugs work in the treatment of lung diseases.
hMSCs are non-hematopoietic, multi-potent progenitor cells with the capacity to generate bone marrow stromal cells as well as adipocytes, chondrocytes, and osteocytes in suitable tissue and other organ sites.
Studying lung stem cells sheds light on the causes of lung disease
A better understanding of lung stem cell and progenitor cell biology can improve our knowledge of how the healthy lung works. This in turn will shed light on the causes of lung diseases such as chronic obstructive pulmonary disease (COPD). Such research could lead to the development of new treatments for lung disease. In fact, lung stem cells may be used in future therapies to repair or regenerate the lungs of patients with severe lung damage or disease.
Current research
Lung stem cells have most frequently been identified and characterized in mice. Studies on mice have allowed researchers to identify the differences between embryonic and adult lung stem cells, discover the role of stem cells in lung repair, and investigate how changes to lung stem cells may lead to lung disease. A current focus of research includes testing if the same stem and progenitor cell populations can be identified in human lungs.
Identifying progenitor and stem cells before and after lung injury
Researchers are also working to determine the role of stem cells in various human lung diseases, including lung cancer and COPD. They have begun examining potential clinical applications of stem cell therapies with several ‘first-in-human’ studies to investigate whether lung stem cells might enhance organ replacement or regeneration in patients.
The future of stem cells in treating lung disease
As researchers continue to improve their understanding of the exact identity and function of human lung stem cells, the potential for clinical applications will be divulged. Researchers will identify methods to control lung stem cells, which can then be tested as treatments for lung diseases. Further research will also investigate the uses of lung stem cells for personalized medicine.
###
- Published in Corporate News / Blog